Joe Paton, head of the Learning Lab at the Champalimaud Neuroscience Programme, has been researching the neurological principles by which the brain gains access to timekeeping, a fundamental dimension of our experience on Earth.
He and his team have narrowed down a population of neurons in the striatum, a deep brain region, that possess a rich capacity to generate time-varying patterns of activity. “You can see this network of neurons in a dish, if you provide some input, there's going to be some reverberation of that input over time,” says Paton. “The patterns of activity that are produced during that reverberation actually provide you information, for instance, about when that input was given.”
Paton’s team ran a set of experiments on mice, training them to distinguish between different intervals of time — like a period of silence between two tones — and whether the interval between the two tones was shorter or longer than 1.5 seconds.
As a result, they were able to show that if you train an animal to make categorical judgments about the duration of some stimulus, that time-neuron pattern of activity systematically evolves more quickly or more slowly according to whether the animal categorizes the interval as having lasted longer or shorter.
So, they asked, if you go in and mess with that neuron pattern, will it mess with that brain’s understanding of time passing? “Understanding how the brain basically orders and positions events in time is really critical for understanding how it learns to guide behavior,” says Paton.
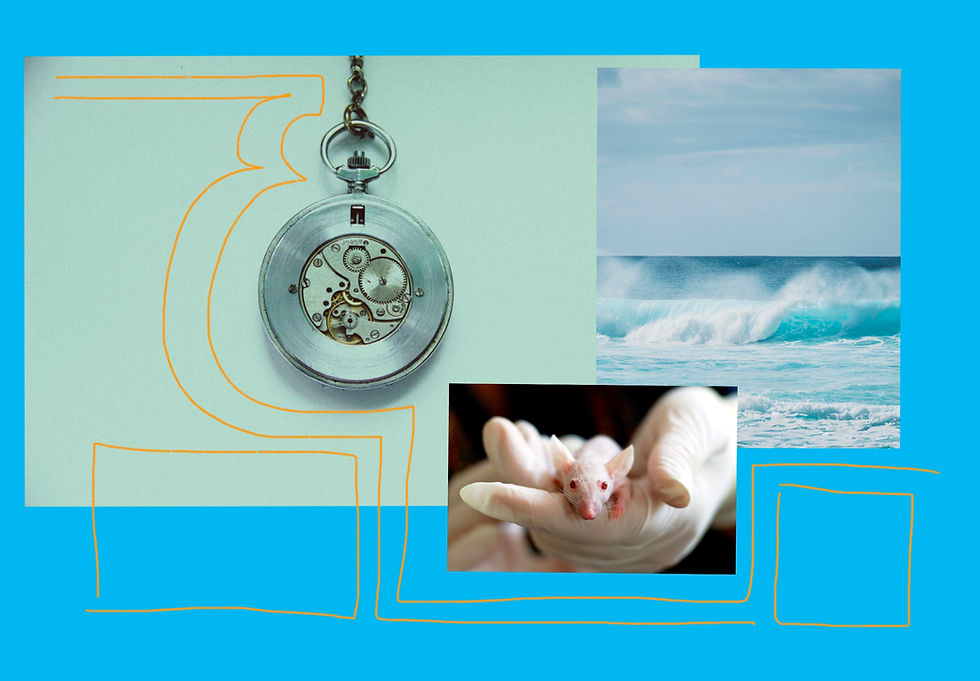
I’m a science journalist, and my work — which has appeared in the New York Times, Guardian, BBC, and National Geographic — is about telling stories of how the mind works. In this Q&A, Paton and I discussed his latest paper in published in scientific journal, Nature in July 2023, and how his team went about looking for the answers to that question.
So, you have this population of neurons and you know, from prior research, that they speed up and slow down in patterns that match the way the brain processes time, but how can you be sure?
Those were just correlation studies, right? You're carefully looking at behavior, quantifying the animal’s sensitivity to time through the judgments it’s making and then you're looking at patterns of activity and you're relating the neural activity to behavior. But of course, correlation doesn't imply causation. To really test whether these patterns of activity are involved in causing the animal's judgments, we need to intervene.
We've got incredible tools nowadays for manipulating neural circuits. What we wanted to do was just keep the pattern of activity the same, but just slow it down or speed it up. So we turned to temperature. In some neural systems, it's been shown that just by changing the temperature of the system, you can slow down or speed up patterns of neural activity while leaving all the structure of that neural activity intact. We designed a thermoelectric device for warming or cooling the striatum focally, while simultaneously recording neural activity, and confirmed that we could use temperature to kind of stretch and contract patterns of neural activity in time.
You can think about networks of interconnected neurons as sort of analogous to a body of water. Just like when you throw a stone into a pool of water, and that causes a bunch of ripples that move over time when you give input to a network of neurons, you'll see patterns of activity moving through the network. What we're doing here with temperature is sort of analogous to making the fluid more viscous or less viscous. So the waves are traveling faster or slower.
Before this study, we didn't really know, conclusively, how the brain was essentially knowing information about when things happen. We didn't know how it gained access to a sense of time.
Since the striatum is in the basal ganglia, did making the rats perceive time as faster or slower also change whether they moved more quickly or more slowly?
Yes, the basal ganglia is a brain system that's implicated in motor control, so that questions makes sense. Dysfunction in the basal ganglia underlies things like Huntington's or Parkinson's diseases, which we think of as motor diseases and movement disorders Some scholars argue that the basal ganglia is involved in learning really detailed moment-by-moment programs for motor control.
If we had effects on the animal’s temporal decisions, we thought we might also see similar effects on the kind of temporal control of their movement. We didn't see that.
Instead, what we saw was that both warming and cooling slowed the animals down slightly. And that's consistent with a long-known role for the basal ganglia in modulating movement vigor. So for instance, in Parkinson's one of the major symptoms is the slowness of movement, and real difficulty with initiating movements.
So these findings indicate that the striatum is critical for deciding ‘what’ to do and ‘when’ to do it, but ‘how’ to do it, and how to control ongoing movement — whether fast or slow — is left to other brain structures.
I know you have some preliminary data that seems to suggest another part of the brain can manipulate the speed and direction at which stuff happens?
Early data suggests that – unlike when we manipulate the temperature in the basal ganglia where we only saw this sort of gain effect on movement — when we do those same kinds of manipulations in the cerebellum part of the brain, we see really striking effects on the continuous control movement. So, not only does motion change in speed, but also direction.
If you imagine you have a series of motor commands that are required for continuously controlling say a reach, if I now take the time base, and I stretch it, you don't just expect that the movement is going to get slower, it’s actually going to follow a different trajectory, because you're going to be issuing those commands for longer. The prediction is you actually make a larger, more eccentric reach. And conversely, when you compress the time base, you expect that you're going to get a less eccentric reach. That's what we see. So seeing this dissociation of roles between two major brain systems is exciting.
Much of this sound quite abstract, how do we make it applicable to our understanding of the real world?
One key application is biology and medicine. These discoveries can provide a more satisfactory understanding of many different disorders that affect the circuits we're studying. I mentioned Parkinson's, and Huntington's, but there are also other diseases that affect the basal ganglia, like obsessive-compulsive disorder (OCD).
We don't usually necessarily think about Parkinson's and OCD as being driven by a similar underlying problem. But in Parkinson's, patients have trouble initiating movements. In OCD, patients have a problem transitioning out of particular patterns of thought. So, could we understand those two diseases as having more in common, and thus could that give rise to more effective interventions? We don't know. That's for future research to try and figure out, but I think it does provide a really attractive organizing principle for what this brain system is doing.